
Supramolecular Synthesis
Background/Motivation
Research in the Supramolecular Synthesis subgroup of the Mirkin group focuses on two main areas: 1) understanding the nuances of small-molecule-induced signal transduction processes in coordination-based complexes and supramolecular assemblies and 2) using coordination-based assembly methodologies to construct abiotic allosteric enzyme mimics.
In 1998, we introduced the Weak-Link Approach (WLA) to supramolecular assembly, a versatile strategy for preparing a wide range of coordination-based systems. The WLA takes advantage of hemilabile ligands, containing comparatively stronger and weaker binding moieties, that can coordinate to metal centers and quantitatively assemble into a single condensed structure (Fig. 1). The WLA yields structures that can be manipulated by exploiting the weaker metal-ligand interactions. For example, small ligating molecules (e.g., Cl–, CO, CH3CN, COO–) can be used to selectively break the weaker metal-ligand bonds, allowing for the conversion of the condensed structure to an open, flexible one (Fig. 2). This process constitutes a form of allosteric control, and over the last more than two decades, we have shown the generality of the WLA for constructing allosteric supramolecular structures, that vary in terms of metal centers used, pocket size and shape, and number of possible accessible states. Moreover, we have demonstrated the WLA as a promising strategy for applications involving stimuli-responsive metallo-supramolecular complexes for guest encapsulation, catalytic and fluorescent molecular tweezers, inorganic light-harvesting systems, multi-state inorganic materials containing regulatory metal nodes, and others.

Figure 1
The crystal structure of a model WLA complex containing a porphyrin photoredox center and a bioinspired light-harvesting antenna. (Adapted from Nat. Commun. 2015, 6, 6541.)
Figure 2
Construction of flexible structures using the WLA.
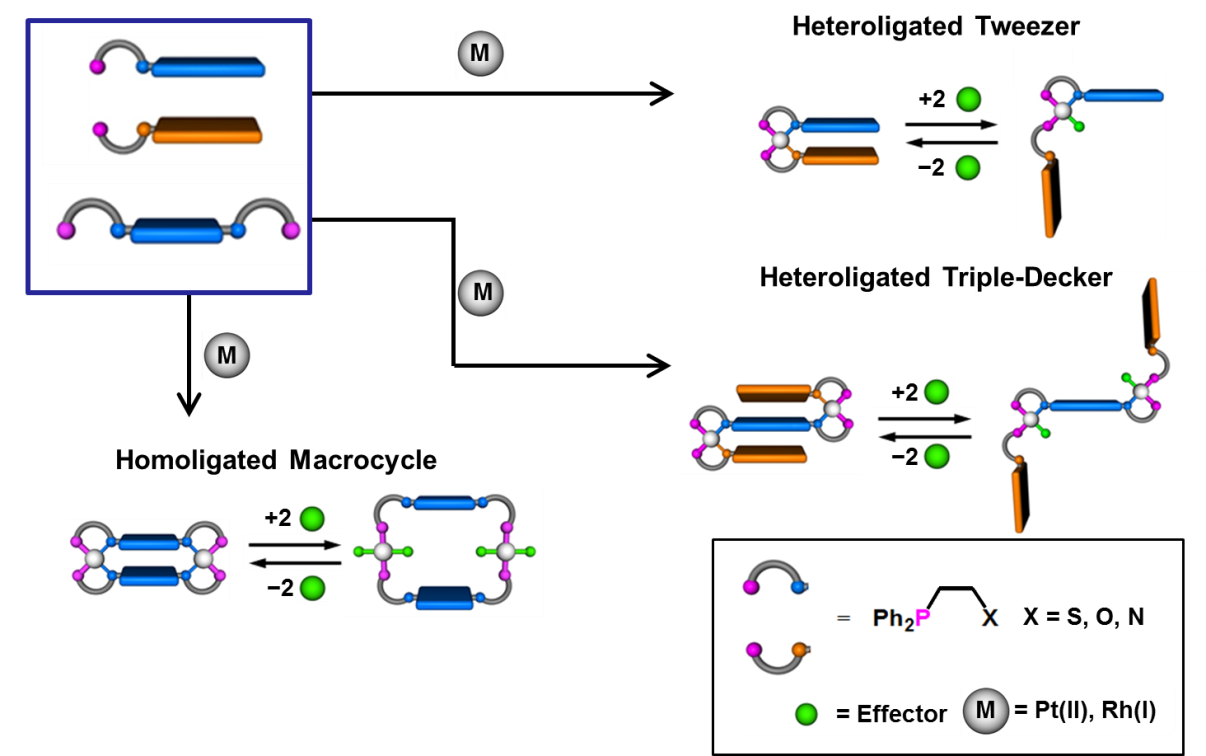
Allosteric Enzyme Mimics Assembled via the Weak-Link Approach and Signal Amplification
Allosteric regulation of enzyme activity often occurs through the binding of a small molecule and/or elemental ion at a secondary site that is not the enzyme’s active site. Unlike other coordination-based assembly methodologies, structures assembled via the WLA can be chemically interconverted between conformational states, allowing one to prepare systems reminiscent of allosteric enzymes. Using the WLA, we prepared the first example of an abiotic, allosterically controllable supramolecular catalyst. A bimetallic Zn(II)-salen macrocyclic complex was constructed wherein the catalyst is ‘off’ in the closed state, but can be activated with the appropriate small molecule effectors and be turned ‘on’ to catalyze an acyl transfer reaction.
Furthermore, the ability to use an external stimulus to trigger a catalytic reaction provides the basis for a general strategy for highly amplified chemical detection systems. In an extension of the aforementioned study based on the Zn(II)-salen macrocyclic systems, we discovered that acetate anions can be detected in a polymerase chain reaction (PCR)-like fashion, resulting in target self-amplification (Fig. 3). In this example the system is coupled to fluorescent indicators, whereby a trace amount of analyte activates a catalytic reaction that generates a fluorescent surrogate for the analyte. This general detection scheme has led to highly sensitive detection methods for various small molecule and elemental ion analytes.
Figure 3
Allosteric supramolecular bimetallic Zn(II) catalyst in the context of acetate target self-amplification in a PCR-like cascade reaction. (Adapted from J. Am. Chem. Soc. 2008, 130, 11590-11591.)

Halide Induced Ligand Rearrangement (HILR)
Our desire to enhance the range of catalytic moieties applicable to WLA architectures prompted the discovery and development of the Halide-Induced Ligand Rearrangement (HILR) reaction, whereby two unique hemilabile ligands can be reacted with a Rh(I) precursor to form the corresponding dissymmetric heteroligated products. We have shown that macrocyclic, tweezer, triple-decker, and other well-defined three-dimensional supramolecular structures can form quantitatively in a “one-pot” synthesis at room temperature. This capability allows one to fine-tune the distances between key units within the targeted structures.
Leveraging the Rh(I)-based HILR reaction, we have developed an allosteric supramolecular triple-layer catalyst used for the ring-opening polymerization of ε-caprolactone (Fig. 4). Using the reversible addition and abstraction of chloride ions, we were able to alter the structure of the complex, moving the bulky blocking groups away from the active site and thus exposing and activating the buried catalyst. This work illustrates our ability to introduce a variety of functional groups into WLA-based, triple-layered structures via coordination chemistry, and it is a powerful and potentially general method for synthesizing protected active centers that can be reversibly activated and deactivated in situ without substantial loss of catalytic activity as a result of cycling.
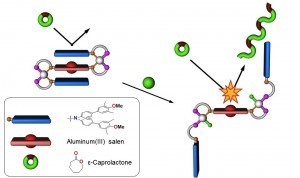
Figure 4
Triple-layer polymerization catalyst synthesized via the WLA and HILR reaction. (Adapted from Science 2010, 330, 66-69.)
Assembly and Allosteric Regulation of Host-Guest Interactions in Molecular Capsules via the Weak-Link Approach
In order to regulate biological functions, such as breathing, protein folding, and signal transduction, enzymes and proteins have evolved advanced active pockets capable of binding substrates with high affinity and specificity. Furthermore, many of these biological hosts will, upon the introduction of an allosteric effector, dramatically change the binding properties of the host structure, enabling the biological entity to reversibly switch between two or more configurations. The unique capability of enzymes to allosterically regulate host-guest properties allows for the existence of complex biological cycles and ensures the realization of homeostasis in organisms. Inspired by the ability of certain enzymatic binding pockets to reversibly switch between multiple guest-binding configurations, we have designed a multi-state allosterically regulated molecular receptor.
In this example, chemical effectors are used to modulate the coordination state of a Pt(II) metal center, which dictates the overall charge and size of the calix[4]arene-based binding pocket in the molecular receptor (Fig. 5). Overall, three configurations are accessible via the WLA: a closed, inactive configuration and two active configurations, semi-open and fully open. The formation of host-guest complexes is only possible in the active configurations since the binding pocket in the closed configuration has completely collapsed. On the other hand, guest selectivity in the active configurations is achieved by controlling the charge and intermolecular interactions between the semi-open and fully open configurations.
Finally, reversibility between the different binding configurations is easily achieved in situ by the simple addition or abstraction of elemental anions from solution. This reversibility allows different guests to occupy the cavity of the molecular receptor. Overall, this work shows how the WLA can be used to assemble biomimetic, supramolecular host architectures with guest-binding properties that can be modulated in a manner reminiscent to those seen in biological systems.
Figure 5
Modulation of host-guest properties in a three-state allosteric molecular receptor via the WLA. (Adapted from J. Am. Chem. Soc. 2014, 136, 10340-10348.)
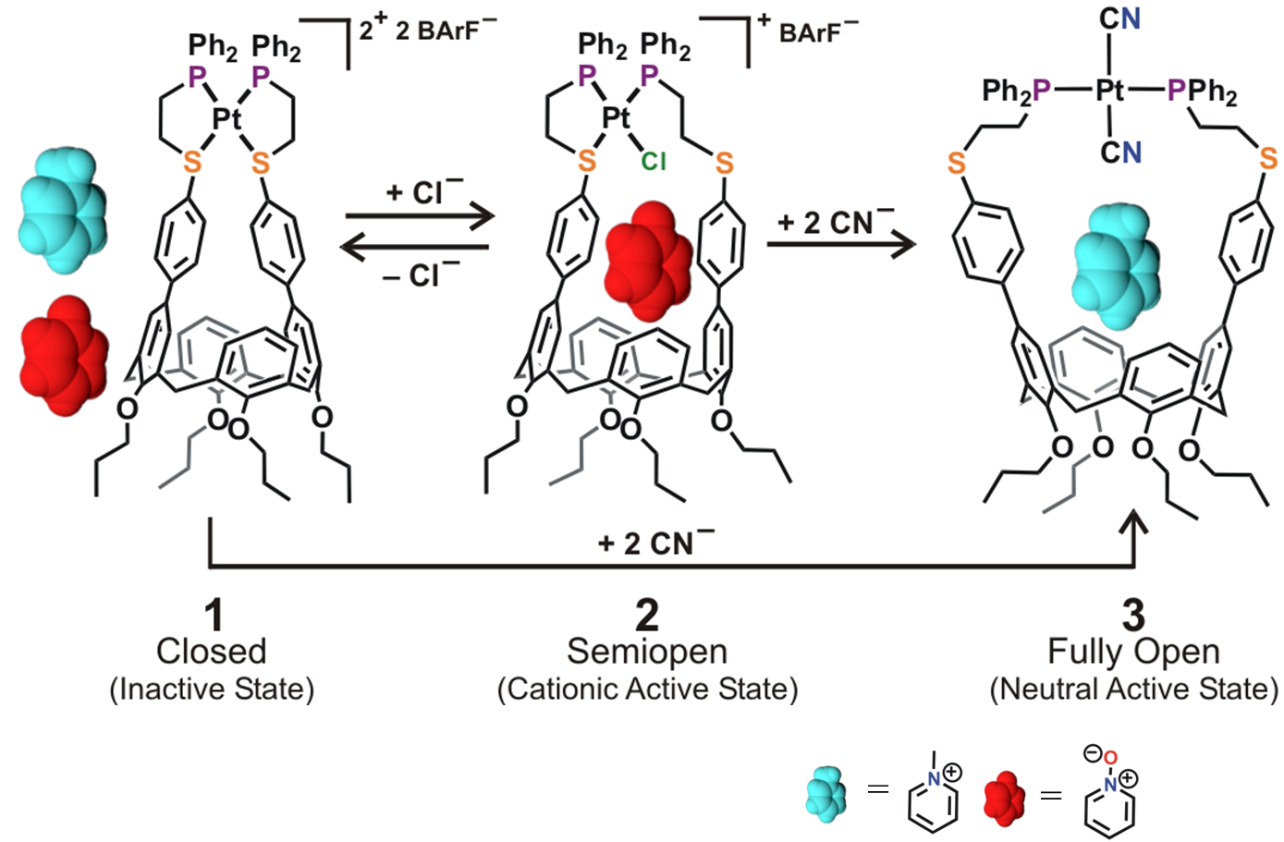
Allosteric Regulation of Photoredox Catalysts and Photochemical Transformations via the Weak-Link Approach
In nature, light harvesting is allosterically regulated via conformational and electronic changes on photosynthetic antenna protein complexes. The allosteric regulation of Photosystem II is important for preventing damage to biological machinery due to overexposure to sunlight and to keep the concentration of ions at healthy levels. Although there are many photoredox complexes, fundamental limitations in current approaches to regulating inorganic light-harvesting mimics prevents their application in fields such as catalysis. We have shown that a light-harvesting antenna/reaction biomimic can be regulated by utilizing a coordination framework incorporating antenna hemilabile ligands that was assembled via the WLA (Fig. 6).
Allosteric regulation is afforded by coupling the conformational changes to the disruptions in the electrochemical landscape of the Rh(I) and organic framework upon recognition of small molecule effectors. The hemilabile ligands enable switching using redox-inactive inputs, such as chloride anions, allowing one to regulate the photoredox catalytic activity of the photosynthetic mimic reversibly and in situ. This assembly shows that bioinspired regulatory structures assembled via the WLA can be utilized as inorganic light-harvesting arrays that display switchable catalytic properties, with potential uses in solar energy conversion and photonic devices.

Figure 6
Regulation of a bioinspired light-harvesting antenna mimic via the WLA. Photocatalytic behavior is controlled via coordination chemistry at a distal, redox-active Rh(I) center. The light-harvesting antenna is shown in green, and the photoredox center is shown in magenta and blue. (Adapted from Nat. Commun. 2015, 6, 6541.)
Allosteric Regulation of Supramolecular Hydrogen-Bonding Oligomers and Catalytic Activity via the Weak-Link Approach
We have demonstrated that the activity of a hydrogen-bond-donating (HBD) catalyst embedded within a coordination framework can be allosterically regulated in situ by controlling oligomerization via simple changes in coordination chemistry at distal Pt(II) nodes (Fig. 7). Using the HILR, a heteroligated Pt(II) triple-decker complex can be formed, which contains a catalytically active diphenylene squaramide moiety and two hydrogen-bond-accepting (HBA) ester moieties. Due to the hemilabile nature of the ligands, the resulting complex can be interconverted between a flexible, semi-open state and a rigid, fully closed state in situ and reversibly.
We confirmed that, in the semi-open state, intermolecular hydrogen-bonding between the HBD and HBA moieties drives oligomerization of the complex and prevents substrate recognition by the catalyst. In the rigid, fully closed state, these interactions are prevented by steric and geometric constraints. Thus, the diphenylene squaramide moiety can catalyze a Friedel–Crafts reaction in the fully closed state, while the semi-open state shows negligible reactivity. This work shows that catalytic activity can be controlled by regulating aggregation through supramolecular conformational changes, a common process observed in living systems, and can be applied to abiotic catalytic frameworks that are relevant to materials synthesis, as well as the detection and amplification of small molecules.
Figure 7
Allosteric regulation of a catalytic squaramide-based triple-decker complex via the WLA. In the flexible semi-open state, self-association via a hydrogen-bond network prevents the squaramide from engaging in catalytic activity. Meanwhile, the rigid conformation of the closed state prevents the formation of oligomers, allowing the substrate to bind the squaramide and initiate the catalytic cycle. (Adapted from J. Am. Chem. Soc. 2014, 136, 16594-16601.)

Redox-Switchable Coordination Complexes
Open coordination sites possess enhanced or unusual reactivities, making them a challenging yet intriguing synthetic target. One strategy we have deployed to tame their reactivity is to mask the open coordination site, which can then be revealed in response to a stimulus. To this end, we designed redox-switchable hemilabile ligands (RHLs) that contain a non-labile heteroatom (strong-link) that anchors the ligand to a metal and a labile heteroatom (weak-link) whose donor strength depends on the ligand oxidation state.
We synthesized a model Pt(II) heteroligated tweezer complex containing an RHL (Fig. 8). In this structure, a ferrocenyl (Fc) moiety is attached to a weak-link thioether. Upon oxidation of Fc, which can be achieved electrochemically or by using chemical oxidants, the Pt–S bond becomes weakened. As a result, exogenous ligands, such as thioanisole (PhSMe) and acetonitrile (MeCN), can displace the -SFc(III) weak-link, bind to the Pt(II) node, and generate a semi-open structure. This structural change is reversible: upon addition of a reducing agent, the fully closed, reduced complex is reformed. These efforts established a strategy for controlling a single coordination site’s occupancy and metal complex architecture using redox chemistry.
Figure 8
A coordination site that is unmasked upon oxidation of the Fc moiety enables control over stoichiometric, exogenous ligand coordination at a Pt(II) metal node. (Adapted from J. Am. Chem. Soc. 2018, 140, 14590–14594.)
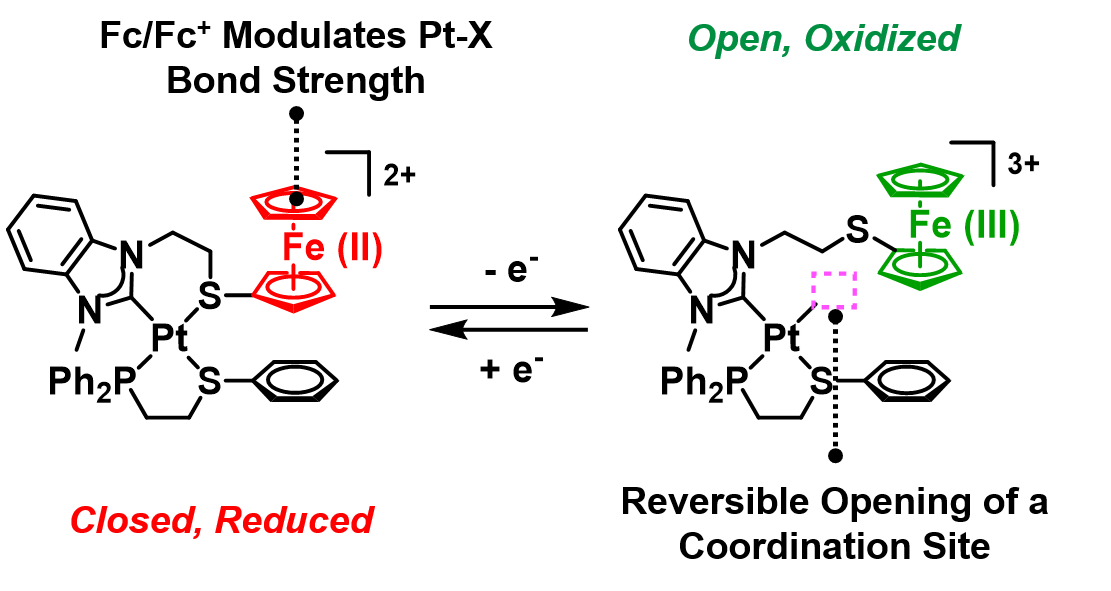
Stimuli-Responsive Molecular Materials and Extended Solids
Addressable, multi-state materials are highly sought after for their potential applications in sensing, imaging, and molecular storage and delivery, but the design of stimuli-responsive materials can often be quite complex because the system must carefully balance factors such as sensitivity to the desired stimulus, degradation of the material over repeated cycles, and effects of the material’s surrounding chemical environment. Furthermore, changing the target stimulus for a material often requires a complete chemical redesign. The WLA is a powerful platform to utilize for material design due to its inherent modularity, sensitivity to a wide range of chemical effectors, and high functional group tolerance. As such, we have been pushing the boundaries of the WLA to synthesize next-generation stimuli-responsive materials.
We began by targeting infinite coordination polymers (ICPs). ICPs are a class of inorganic solids in which multiple metal ions are bridged by repeated, multi-functional organic ligands to form extended 1D, 2D, or 3D solids. We have utilized the control over molecular geometry and flexibility afforded by the WLA to synthesize a series of stimuli-responsive ICP particles. The particles feature WLA nodes containing hemilabile ligands with appended terpyridine (TPy) moieties bound to either Pt(II) or Pd(II) (Fig. 9). Polymerization of the WLA nodes occurs after reaction of Fe(II) with TPy groups. Particles synthesized from Pt(II)-based subunits are spherical aggregates or particles with rough surfaces based on the structural state of the WLA node, whereas particles synthesized from Pd(II) subunits are smooth and well-formed in both structural states. This was attributed to the much faster Pd-ligand exchange rate versus that of the Pt-based analogue, illustrating the impact of the molecular subunits’ ability to rearrange particle morphology. From this work, we were able to show that ICP particle morphology can be controlled via the use of stimuli-responsive subunits.
Stimuli-responsive photoluminescent materials have been highly sought after due to their applications in sensing and imaging. We targeted a WLA complex in which the emission properties of two fluorophores could be toggled via a structural change or phase transition. To this end, we synthesized a heteroligated, WLA Pt(II) complex featuring hemilabile ligands with either a bound pyrene or tetraphenylethene (TPE) moiety and compared the emission properties of both the semi-open and fully closed forms in solution and in the solid state (Fig. 10). In both the semi-open and fully closed forms, we found enhanced emission in the solid state due to the increased emission of the TPE moiety and quenching of the pyrene. Furthermore, opening the fully closed complex to the semi-open complex via chloride binding resulted in a 9.6% increase in quantum yield. We postulated that this increase resulted from an increase in distance between the Pt(II) center and the pyrene moiety, suppressing the distance-dependent heavy-atom effect. With this work, we introduced a new platform for synthesizing multi-state, stimuli-responsive systems with tunable emission pathways and fluorescence outputs. Importantly, the modularity of the WLA allows these complexes to be incorporated to larger, supramolecular constructs.

Figure 9
Structural regulation in the WLA subunits upon chloride ion binding induces a change in ICP particle morphology. For the Pt(II) ICP particles, chloride ion binding to form the semi-open state results in 200-500 nm spherical particles with rough surfaces, whereas when the WLA node was closed, only aggregates were formed. (Adapted from Chem. Mater. 2017, 29, 10284-10288.)
Figure 10
A coordination site that is unmasked upon oxidation of the Fc moiety enables control over stoichiometric, exogenous ligand coordination at a Pt(II) metal node. (Adapted from J. Am. Chem. Soc. 2018, 140, 14590–14594.)

Supramolecular Synthesis Subgroup Members
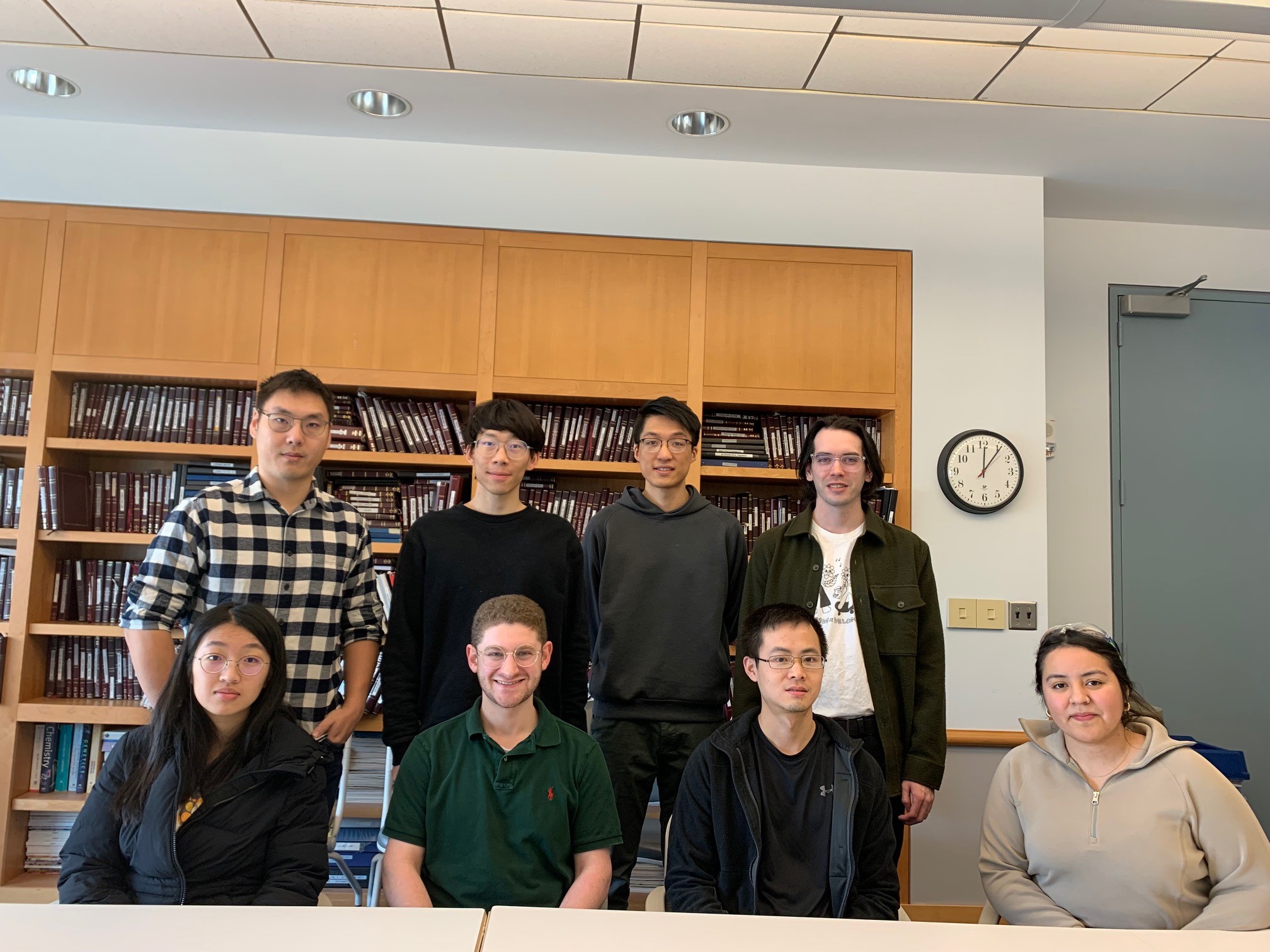
Back Row (L-R): Yiming Hu (subgroup leader), Cuizheng Zhang, Yi Xie, Peter Smith
Front Row (L-R): Fiona Wang, Adam Claman, Henry Han (subgroup leader) Jennifer Delgado